LTC1735IS Linear Technology, LTC1735IS Datasheet - Page 21
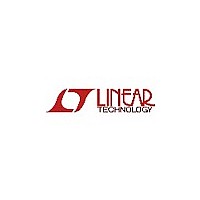
LTC1735IS
Manufacturer Part Number
LTC1735IS
Description
IC REG SW STEP-DOWN SYNC 16-SOIC
Manufacturer
Linear Technology
Type
Step-Down (Buck)r
Datasheet
1.LTC1735CSPBF.pdf
(32 pages)
Specifications of LTC1735IS
Internal Switch(s)
No
Synchronous Rectifier
Yes
Number Of Outputs
1
Voltage - Output
0.8 ~ 6 V
Current - Output
3A
Frequency - Switching
300kHz
Voltage - Input
4 ~ 30 V
Operating Temperature
-40°C ~ 85°C
Mounting Type
Surface Mount
Package / Case
16-SOIC (3.9mm Width)
Lead Free Status / RoHS Status
Contains lead / RoHS non-compliant
Power - Output
-
Available stocks
Company
Part Number
Manufacturer
Quantity
Price
Company:
Part Number:
LTC1735IS
Manufacturer:
Linear Technology
Quantity:
135
Part Number:
LTC1735IS
Manufacturer:
LT/凌特
Quantity:
20 000
Company:
Part Number:
LTC1735IS#PBF
Manufacturer:
LT
Quantity:
890
Part Number:
LTC1735IS-1
Manufacturer:
LINEAR/凌特
Quantity:
20 000
APPLICATIO S I FOR ATIO
Although all dissipative elements in the circuit produce
losses, 4 main sources usually account for most of the
losses in LTC1735 circuits: 1) V
current, 3) I
losses.
1) The V
electrical characteristics which excludes MOSFET driver
and control currents. V
loss that increases with V
2) INTV
control currents. The MOSFET driver current results from
switching the gate capacitance of the power MOSFETs.
Each time a MOSFET gate is switched from low to high to
low again, a packet of charge dQ moves from INTV
ground. The resulting dQ/dt is a current out of INTV
is typically much larger than the control circuit current. In
continuous mode, I
are the gate charges of the topside and bottom-side
MOSFETs.
Supplying INTV
from an output-derived or other high efficiency source will
scale the V
circuits by a factor of (Duty Cycle)/(Efficiency). For ex-
ample, in a 20V to 5V application, 10mA of INTV
results in approximately 3mA of V
the mid-current loss from 10% or more (if the driver was
powered directly from V
3) I
MOSFET, inductor and current shunt. In continuous mode
the average output current flows through L and R
but is “chopped” between the topside main MOSFET and
the synchronous MOSFET. If the two MOSFETs have
approximately the same R
one MOSFET can simply be summed with the resistances
of L and R
R
the total resistance is 0.09 . This results in losses ranging
from 2% to 9% as the output current increases from 1A to
5A for a 5V output, or a 3% to 14% loss for a 3.3V output.
Effeciency varies as the inverse square of V
same external components and output power level. I
losses cause the efficiency to drop at high output currents.
DS(ON)
2
R losses are predicted from the DC resistances of the
CC
= 0.03 , R
IN
SENSE
current is the sum of the MOSFET driver and
IN
current is the DC supply current given in the
2
current required for the driver and control
R losses, 4) Topside MOSFET transition
CC
to obtain I
power through the EXTV
GATECHG
U
L
= 0.05 and R
IN
current results in a small (<0.1%)
IN
IN
U
) to only a few percent.
2
DS(ON)
R losses. For example, if each
.
= f(Q
T
, then the resistance of
IN
+Q
IN
W
current. This reduces
SENSE
B
current, 2) INTV
), where Q
CC
= 0.01 , then
switch input
OUT
CC
U
T
current
and Q
for the
CC
SENSE
CC
that
2
CC
to
R
B
,
4) Transition losses apply only to the topside MOSFET(s)
and only become significant when operating at high input
voltages (typically 12V or greater). Transition losses can
be estimated from:
Other “hidden” losses such as copper trace and internal
battery resistances can account for an additional 5% to
10% efficiency degradation in portable systems. It is very
important to include these “system” level losses in the
design of a system. The internal battery and fuse resis-
tance losses can be minimized by making sure that C
adequate charge storage and very low ESR at the switch-
ing frequency. A 25W supply will typically require a
minimum of 20 F to 40 F of capacitance having a maxi-
mum of 0.01 to 0.02 of ESR. Other losses including
Schottky conduction losses during dead-time and induc-
tor core losses generally account for less than 2% total
additional loss.
Checking Transient Response
The regulator loop response can be checked by looking at
the load current transient response. Switching regulators
take several cycles to respond to a step in load current.
When a load step occurs, V
to I
tance of C
C
regulator to adapt to the current change and return V
to its steady-state value. During this recovery time V
can be monitored for excessive overshoot or ringing,
which would indicate a stability problem. OPTI-LOOP
compensation allows the transient response to be opti-
mized over a wide range of output capacitance and ESR
values. The availability of the I
optimization of control loop behavior but also provides a
DC coupled and AC filtered closed loop response test
point. The DC step, rise time and settling at this test point
truly reflects the closed loop response. Assuming a pre-
dominantly second order system, phase margin and/or
damping factor can be estimated using the percentage of
overshoot seen at this pin. The bandwidth can also be
estimated by examining the rise time at the pin. The I
external components shown in the Figure 1 circuit will
provide an adequate starting point for most applications.
OUT
Transition Loss = (1.7) V
LOAD
, generating the feedback error signal that forces the
OUT
(ESR), where ESR is the effective series resis-
. I
LOAD
also begins to charge or discharge
OUT
IN
2
shifts by an amount equal
I
O(MAX)
TH
pin not only allows
C
LTC1735
RSS
f
21
IN
1735fc
has
OUT
OUT
TH