CS51311GD14 Cherry Semiconductor Corporation, CS51311GD14 Datasheet - Page 13
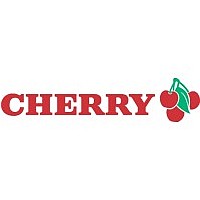
CS51311GD14
Manufacturer Part Number
CS51311GD14
Description
Synchronous CPU Buck Controller for 12V and 5V Applications
Manufacturer
Cherry Semiconductor Corporation
Datasheet
1.CS51311GD14.pdf
(19 pages)
Once the total ESR of the input capacitors is known, the
input capacitor ripple voltage can be determined using the
formula:
where
The designer must determine the input capacitor power
loss in order to ensure there isn’t excessive power dissipa-
tion through these components. The following formula is
used:
where
Step 6: Selection of the Input Inductor
A CPU switching regulator, such as the one in a buck
topology, must not disturb the primary +5V supply. One
method of achieving this is by using an input inductor and
a bypass capacitor. The input inductor isolates the +5V
supply from the noise generated in the switching portion
of the microprocessor buck regulator and also limits the
inrush current into the input capacitors upon power up.
The inductor’s limiting effect on the input current slew rate
becomes increasingly beneficial during load transients. The
worst case is when the CPU load changes from no load to
full load (load step), a condition under which the highest
voltage change across the input capacitors is also seen by
the input inductor. The inductor successfully blocks the
ripple current while placing the transient current require-
ments on the input bypass capacitor bank, which has to
initially support the sudden load change.
The minimum inductance value for the input inductor is
therefore:
where
The designer must select the LC filter pole frequency so
that at least 40dB attenuation is obtained at the regulator
switching frequency. The LC filter is a double-pole net-
work with a slope of −2, a roll-off rate of –40dB/dec, and a
corner frequency:
where
V
I
ESR
P
I
ESR
L
∆V = voltage seen by the input inductor during a full
load swing;
(dI/dt)
rate (0.1A/µs for a Pentium® II power supply).
L = input inductor;
C = input capacitor(s).
CIN(RMS)
CIN(RMS)
CIN(RMS)
IN
CIN(RMS)
= input inductor value;
CIN
CIN
MAX
= total input capacitor ESR.
= total input capacitor ESR.
= total input RMS current;
= total input RMS current;
= input capacitor RMS power dissipation;
= input capacitor RMS voltage;
= maximum allowable input current slew
V
P
CIN(RMS)
CIN(RMS)
L
IN
f
=
C
= I
= I
=
CIN(RMS)
(dI/dt)
CIN(RMS)
2π LC
∆V
1
MAX
2
× ESR
,
× ESR
,
CIN
Application Information: continued
CIN
,
13
Step 7: Selection of the Switching FET
FET Basics
The use of the MOSFET as a power switch is propelled by
two reasons: 1) Its very high input impedance; and 2) Its very
fast switching times. The electrical characteristics of a MOS-
FET are considered to be those of a perfect switch. Control
and drive circuitry power is therefore reduced. Because the
input impedance is so high, it is voltage driven. The input
of the MOSFET acts as if it were a small capacitor, which
the driving circuit must charge at turn on. The lower the
drive impedance, the higher the rate of rise of V
faster the turn- on time. Power dissipation in the switching
MOSFET consists of 1) conduction losses, 2) leakage losses,
3) turn-on switching losses, 4) turn-off switching losses,
and 5) gate-transitions losses. The latter three losses are
proportional to frequency. For the conducting power dissi-
pation rms values of current and resistance are used for
true power calculations. The fast switching speed of the
MOSFET makes it indispensable for high-frequency power
supply applications. Not only are switching power losses
minimized, but also the maximum usable switching fre-
quency is considerably higher. Switching time is indepen-
dent of temperature. Also, at higher frequencies, the use of
smaller and lighter components (transformer, filter choke,
filter capacitor) reduces overall component cost while
using less space for more efficient packaging at lower
weight.
The MOSFET has purely capacitive input impedance. No
DC current is required. It is important to keep in mind the
drain current of the FET has a negative temperature coeffi-
cient. Increase in temperature causes higher on-resistance
and greater leakage current. For switching circuits, V
should be low to minimize power dissipation at a given I
and V
switching times are determined by device capacitance,
stray capacitance, and the impedance of the gate drive cir-
cuit. Thus the gate driving circuit must have high momen-
tary peak current sourcing and sinking capability for
switching the MOSFET. The input capacitance, output
capacitance and reverse-transfer capacitance also increase
with increased device current rating.
Two considerations complicate the task of estimating
switching times. First, since the magnitude of the input
capacitance, C
determined by the gate-drive impedance and C
during the switching cycle. Consequently, computation of
the rise time of the gate voltage by using a specific gate-
drive impedance and input capacitance yields only a rough
estimate. The second consideration is the effect of the
“Miller” capacitance, C
the following discussion. For example, when a device is on,
V
charged to V
the drain is considered the positive electrode. When the
drain is “off”, C
In this case the voltage across C
the potential from gate-to-source is near zero volts and V
is essentially the drain supply voltage. During turn-on and
turn-off, these large swings in gate-to-drain voltage tax the
current sourcing and sinking capabilities of the gate drive.
In addition to charging and discharging C
must also supply the displacement current required by
DS(ON)
GS
is fairly small and V
should be high to accomplish this. MOSFET
DS(ON)
ISS
DG
, varies with V
is charged to quite a different potential.
− V
GS
RSS
, which is a negative potential if
, which is referred to as C
GS
is about 12V. C
DS
DG
, the RC time constant
is a positive value since
GS
, the gate drive
DG
ISS
GS
is
, and the
changes
DG
DS(ON)
in
D
DS
,