MAX1714AEEP Maxim Integrated Products, MAX1714AEEP Datasheet - Page 18
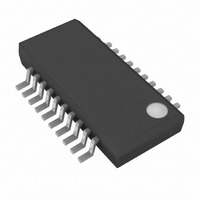
MAX1714AEEP
Manufacturer Part Number
MAX1714AEEP
Description
IC CTRLR HS STEPDOWN 20-QSOP
Manufacturer
Maxim Integrated Products
Type
Step-Down (Buck)r
Datasheet
1.MAX1714AEEP.pdf
(24 pages)
Specifications of MAX1714AEEP
Internal Switch(s)
No
Synchronous Rectifier
Yes
Number Of Outputs
1
Voltage - Output
2.5V, 3.3V, Adj
Current - Output
8A
Frequency - Switching
600kHz
Voltage - Input
2 ~ 28 V
Operating Temperature
-40°C ~ 85°C
Mounting Type
Surface Mount
Package / Case
20-QSOP
Power - Output
727mW
Output Voltage
1 V to 5.5 V
Output Current
3000 mA
Mounting Style
SMD/SMT
Switching Frequency
600 KHz
Maximum Operating Temperature
+ 85 C
Minimum Operating Temperature
- 40 C
Synchronous Pin
No
Topology
Buck
Case
SSOP20
Dc
04+
Lead Free Status / RoHS Status
Contains lead / RoHS non-compliant
Available stocks
Company
Part Number
Manufacturer
Quantity
Price
Part Number:
MAX1714AEEP
Manufacturer:
MAXIM/美信
Quantity:
20 000
Company:
Part Number:
MAX1714AEEP+
Manufacturer:
Maxim Integrated Products
Quantity:
135
Company:
Part Number:
MAX1714AEEP+
Manufacturer:
ISSI
Quantity:
7 229
Part Number:
MAX1714AEEP+
Manufacturer:
MAXIM/美信
Quantity:
20 000
Company:
Part Number:
MAX1714AEEP+T
Manufacturer:
MAXM
Quantity:
7 341
Company:
Part Number:
MAX1714AEEP-T
Manufacturer:
ISSI
Quantity:
6 816
Part Number:
MAX1714AEEP-T
Manufacturer:
MAXIM/美信
Quantity:
20 000
For optimal circuit reliability, choose a capacitor that
has less than 10°C temperature rise at the peak ripple
current.
Most of the following MOSFET guidelines focus on the
challenge of obtaining high load-current capability (>5A)
when using high-voltage (>20V) AC adapters. Low-cur-
rent applications usually require less attention.
For maximum efficiency, choose a high-side MOSFET
(Q1) that has conduction losses equal to the switching
losses at the optimum battery voltage (15V). Check to
ensure that the conduction losses at minimum input
voltage don’t exceed the package thermal limits or
violate the overall thermal budget. Check to ensure that
conduction losses plus switching losses at the maxi-
mum input voltage don’t exceed the package ratings or
violate the overall thermal budget.
Choose a low-side MOSFET (Q2) that has the lowest
possible R
age (i.e., SO-8), and is reasonably priced. Ensure that
the MAX1714 DL gate driver can drive Q2; in other
words, check that the gate isn’t pulled up by the high-
side switch turn on, due to parasitic drain-to-gate capac-
itance, causing cross-conduction problems. Switching
losses aren’t an issue for the low-side MOSFET, since it’s
a zero-voltage switched device when used in the buck
topology.
Worst-case conduction losses occur at the duty factor
extremes. For the high-side MOSFET, the worst-case
power dissipation due to resistance occurs at minimum
battery voltage:
Generally, a small high-side MOSFET is desired to
reduce switching losses at high input voltages. However,
the R
pation limits often limits how small the MOSFET can be.
Again, the optimum occurs when the switching (AC)
losses equal the conduction (R
switching losses don’t usually become an issue until the
input is greater than approximately 15V.
Switching losses in the high-side MOSFET can become
an insidious heat problem when maximum AC adapter
voltages are applied, due to the squared term in the
CV
you’ve chosen for adequate R
ages becomes extraordinarily hot when subjected to
V
Calculating the power dissipation in Q1 due to switching
losses is difficult, since it must allow for difficult-to-quanti-
High-Speed Step-Down Controller
for Notebook Computers
18
PD(Q1 Resistive) = (V
IN(MAX)
2
F switching loss equation. If the high-side MOSFET
______________________________________________________________________________________
DS(ON)
, you must reconsider your choice of MOSFET.
DS(ON)
required to stay within package power-dissi-
, comes in a moderate to small pack-
MOSFET Power Dissipation
OUT
Power MOSFET Selection
/ V
IN(MIN)
DS(ON)
DS(ON)
) · I
) losses. High-side
at low battery volt-
LOAD 2
· R
DS(ON)
fy factors that influence the turn-on and turn-off times.
These factors include the internal gate resistance, gate
charge, threshold voltage, source inductance, and PC
board layout characteristics. The following switching loss
calculation provides only a very rough estimate and is no
substitute for breadboard evaluation, preferably including
a sanity check using a thermocouple mounted on Q1.
where C
and I
typical).
For the low-side MOSFET, Q2, the worst-case power dis-
sipation always occurs at maximum battery voltage:
The absolute worst case for MOSFET power dissipation
occurs under heavy overloads that are greater than
I
current limit and cause the fault latch to trip. To protect
against this possibility, you must “overdesign” the circuit
to tolerate I
where I
by the current-limit circuit, including threshold tolerance
and on-resistance variation. This means that the
MOSFETs must be very well heatsinked. If short-circuit
protection without overload protection is enough, a nor-
mal I
stresses.
Choose a Schottky diode D1 having a forward voltage
low enough to prevent the Q2 MOSFET body diode from
turning on during the dead time. As a general rule, a
diode having a DC current rating equal to 1/3 of the load
current is sufficient. This diode is optional, and if efficien-
cy isn’t critical it can be removed.
The output voltage adjust range for continuous-conduc-
tion operation is restricted by the nonadjustable 500ns
(max) minimum off-time one-shot. For best dropout per-
formance, use the slowest (200kHz) on-time setting.
When working with low input voltages, the duty-factor
limit must be calculated using worst-case values for on-
and off-times. Manufacturing tolerances and internal
propagation delays introduce an error to the TON K-fac-
tor. This error is greater at higher frequencies (Table 5).
Also, keep in mind that transient response performance
of buck regulators operated close to dropout is poor,
LOAD(MAX)
PD(Q2) = (1 - V
PD(Q1 switching)
LOAD
GATE
LIMIT(HIGH)
RSS
is the peak gate-drive source/sink current (1A
LOAD
value can be used for calculating component
but are not quite high enough to exceed the
is the reverse transfer capacitance of Q1
= I
OUT
is the maximum valley current allowed
LIMIT(HIGH)
=
/ V
C
IN(MAX)
RSS
Application Issues
Dropout Performance
⋅
+ [(LIR / 2) · I
V
IN(MAX)
) · I
I
GATE
LOAD 2
2
⋅ ⋅
f I
· R
LOAD(MAX)
LOAD
DS(ON)
],