ACPL-C797-500E Avago Technologies US Inc., ACPL-C797-500E Datasheet - Page 14
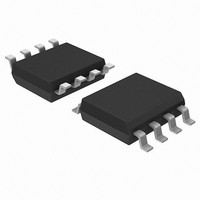
ACPL-C797-500E
Manufacturer Part Number
ACPL-C797-500E
Description
Isolated Sigma-Delta, T/R+IEC+LF
Manufacturer
Avago Technologies US Inc.
Series
-r
Type
Sigma-Delta Modulatorr
Datasheet
1.ACPL-C797-000E.pdf
(16 pages)
Specifications of ACPL-C797-500E
Voltage - Isolation
5000Vrms
Input Type
DC
Voltage - Supply
3 V ~ 5.5 V, 4.5 V ~ 5.5 V
Operating Temperature
-40°C ~ 105°C
Mounting Type
Surface Mount
Package / Case
8-SOIC (0.268", 6.81mm Width)
Lead Free Status / Rohs Status
Lead free / RoHS Compliant
Available stocks
Company
Part Number
Manufacturer
Quantity
Price
Part Number:
ACPL-C797-500E
Manufacturer:
AVAGO/安华高
Quantity:
20 000
PC Board Layout
The design of the printed circuit board (PCB) should follow
good layout practices, such as keeping bypass capacitors
close to the supply pins, keeping output signals away from
input signals, the use of ground and power planes, etc. In
addition, the layout of the PCB can also affect the isolation
transient immunity (CMR) of the isolated modulator, due
primarily to stray capacitive coupling between the input
and the output circuits. To obtain optimal CMR perfor-
mance, the layout of the PC board should minimize any
stray coupling by maintaining the maximum possible
distance between the input and output sides of the circuit
and ensuring that any ground or power plane on the PC
board does not pass directly below or extend much wider
than the body of the isolated modulator.
Shunt Resistors
The current-sensing shunt resistor should have low re-
sistance (to minimize power dissipation), low inductance
(to minimize di/dt induced voltage spikes which could
adversely affect operation), and reasonable tolerance (to
maintain overall circuit accuracy). Choosing a particu-
lar value for the shunt is usually a compromise between
minimizing power dissipation and maximizing accuracy.
Smaller shunt resistances decrease power dissipation,
while larger shunt resistances can improve circuit accuracy
by utilizing the full input range of the isolated modulator.
The first step in selecting a shunt is determining how
much current the shunt will be sensing. The graph in
Figure 20 shows the RMS current in each phase of a three-
phase induction motor as a function of average motor
output power (in horsepower, hp) and motor drive supply
voltage. The maximum value of the shunt is determined
by the current being measured and the maximum rec-
ommended input voltage of the isolated modulator. The
maximum shunt resistance can be calculated by taking the
maximum recommended input voltage and dividing by
the peak current that the shunt should see during normal
operation. For example, if a motor will have a maximum
Figure 20. Motor Output Horsepower vs. Motor Phase Current and Supply
14
40
35
30
25
20
15
10
5
0
0
5
440 V
380 V
220 V
120 V
MOTOR PHASE CURRENT - A (rms)
10
15
20
25
30
35
RMS current of 10 A and can experience up to 50%
overloads during normal operation, then the peak current
is 21.1 A (= 10 × 1.414 × 1.5). Assuming a maximum input
voltage of 200 mV, the maximum value of shunt resistance
in this case would be about 10 m:.
The maximum average power dissipation in the shunt
can also be easily calculated by multiplying the shunt re-
sistance times the square of the maximum RMS current,
which is about 1 W in the previous example.
If the power dissipation in the shunt is too high, the resis-
tance of the shunt can be decreased below the maximum
value to decrease power dissipation. The minimum value
of the shunt is limited by precision and accuracy require-
ments of the design. As the shunt value is reduced, the
output voltage across the shunt is also reduced, which
means that the offset and noise, which are fixed, become
a larger percentage of the signal amplitude. The selected
value of the shunt will fall somewhere between the
minimum and maximum values, depending on the par-
ticular requirements of a specific design.
When sensing currents large enough to cause signifi-
cant heating of the shunt, the temperature coefficient
(tempco) of the shunt can introduce nonlinearity due to
the signal dependent temperature rise of the shunt. The
effect increases as the shunt-to-ambient thermal resis-
tance increases. This effect can be minimized either by
reducing the thermal resistance of the shunt or by using
a shunt with a lower tempco. Lowering the thermal resis-
tance can be accomplished by repositioning the shunt
on the PC board, by using larger PC board traces to carry
away more heat, or by using a heat sink.
For a two-terminal shunt, as the value of shunt resistance
decreases, the resistance of the leads becomes a signifi-
cant percentage of the total shunt resistance. This has two
primary effects on shunt accuracy. First, the effective resis-
tance of the shunt can become dependent on factors such
as how long the leads are, how they are bent, how far they
are inserted into the board, and how far solder wicks up
the lead during assembly (these issues will be discussed
in more detail shortly). Second, the leads are typically
made from a material such as copper, which has a much
higher tempco than the material from which the resistive
element itself is made, resulting in a higher tempco for the
shunt overall. Both of these effects are eliminated when a
four-terminal shunt is used. A four-terminal shunt has two
additional terminals that are Kelvin-connected directly
across the resistive element itself; these two terminals are
used to monitor the voltage across the resistive element
while the other two terminals are used to carry the load
current. Because of the Kelvin connection, any voltage
drops across the leads carrying the load current should
have no impact on the measured voltage.