HCNR201-300E Avago Technologies US Inc., HCNR201-300E Datasheet - Page 18
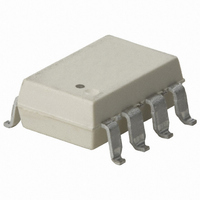
HCNR201-300E
Manufacturer Part Number
HCNR201-300E
Description
OPTOCOUPLER ANLG DC-1MHZ GW 8SMD
Manufacturer
Avago Technologies US Inc.
Specifications of HCNR201-300E
Output Type
Linear Photovoltaic
Input Type
DC
Package / Case
8-SMD Gull Wing
Number Of Channels
1
Voltage - Isolation
5000Vrms
Current Transfer Ratio (min)
0.36% @ 10mA
Current Transfer Ratio (max)
0.72% @ 10mA
Current - Dc Forward (if)
25mA
Mounting Type
Surface Mount, Gull Wing
Current Transfer Ratio
0.36 % to 0.72 %
Forward Current
1 mA to 20 mA
Isolation Voltage
5000 Vrms
Minimum Forward Diode Voltage
1.2 V
Output Device
Photodiode
Configuration
1 Channel
Maximum Forward Diode Voltage
1.95 V
Maximum Reverse Diode Voltage
2.5 V
Maximum Input Diode Current
25 mA
Maximum Power Dissipation
60 mW
Maximum Operating Temperature
+ 100 C
Minimum Operating Temperature
- 55 C
No. Of Channels
1
Optocoupler Output Type
Photodiode
Input Current
20mA
Output Voltage
15V
Opto Case Style
SMD
No. Of Pins
8
Forward Voltage
1.6V
Rohs Compliant
Yes
Forward Current If
25mA
Lead Free Status / RoHS Status
Lead free / RoHS Compliant
Voltage - Output
-
Current - Output / Channel
-
Vce Saturation (max)
-
Lead Free Status / Rohs Status
Lead free / RoHS Compliant
Other names
516-1609-5
Available stocks
Company
Part Number
Manufacturer
Quantity
Price
Company:
Part Number:
HCNR201-300E
Manufacturer:
AVAGO
Quantity:
30 000
The preceding circuits were presented to illustrate the
flexibility in designing analog isolation circuits using the
HCNR200/201. The next section presents several com‑
plete schematics to illustrate practical applications of the
HCNR200/201.
Example Application Circuits
The circuit shown in Figure 16 is a high‑speed low‑cost
circuit designed for use in the feedback path of switch‑
mode power supplies. This application requires good
bandwidth, low cost and stable gain, but does not re‑
quire very high accuracy. This circuit is a good example
of how a designer can trade off accuracy to achieve
improvements in bandwidth and cost. The circuit has a
bandwidth of about 1.5 MHz with stable gain character‑
istics and requires few external components.
Although it may not appear so at first glance, the circuit
in Figure 16 is essentially the same as the circuit in Fig‑
ure 12a. Amplifier A1 is comprised of Q1, Q2, R3 and R4,
while amplifier A2 is comprised of Q3, Q4, R5, R6 and R7.
The circuit operates in the same manner as well; the only
difference is the performance of amplifiers A1 and A2.
The lower gains, higher input currents and higher offset
voltages affect the accuracy of the circuit, but not the
way it operates. Because the basic circuit operation has
not changed, the circuit still has good gain stability. The
use of discrete transistors instead of op‑amps allowed
the design to trade off accuracy to achieve good band‑
width and gain stability at low cost.
To get into a little more detail about the circuit, R1 is se‑
lected to achieve an LED current of about 7‑10 mA at the
nominal input operating voltage according to the fol‑
lowing equation:
where K
0.5%. R2 is then selected to achieve the desired output
voltage according to the equation,
The purpose of R4 and R6 is to improve the dynamic re‑
sponse (i.e., stability) of the input and output circuits by
lowering the local loop gains. R3 and R5 are selected to
provide enough current to drive the bases of Q2 and Q4.
And R7 is selected so that Q4 operates at about the same
collector current as Q2.
The next circuit, shown in Figure 17, is designed to achieve
the highest possible accuracy at a reasonable cost. The
high accuracy and wide dynamic range of the circuit is
achieved by using low‑cost precision op‑amps with very
low input bias currents and offset voltages and is limited
by the performance of the optocoupler. The circuit is de‑
signed to operate with input and output voltages from
1 mV to 10 V.
18
1
I
V
(i.e., I
F
OUT
= (V
/V
IN
PD1
IN
/R1)/K1,
= R2/R1.
/I
F
) of the optocoupler is typically about
The circuit operates in the same way as the others. The
only major differences are the two compensation capaci‑
tors and additional LED drive circuitry. In the high‑speed
circuit discussed above, the input and output circuits are
stabilized by reducing the local loop gains of the input
and output circuits. Because reducing the loop gains
would decrease the accuracy of the circuit, two compen‑
sation capacitors, C1 and C2, are instead used to improve
circuit stability. These capacitors also limit the bandwidth
of the circuit to about 10 kHz and can be used to reduce
the output noise of the circuit by reducing its bandwidth
even further.
The additional LED drive circuitry (Q1 and R3 through
R6) helps to maintain the accuracy and bandwidth of the
circuit over the entire range of input voltages. Without
these components, the transconductance of the LED
driver would decrease at low input voltages and LED
currents. This would reduce the loop gain of the input
circuit, reducing circuit accuracy and bandwidth. D1 pre‑
vents excessive reverse voltage from being applied to
the LED when the LED turns off completely.
No offset adjustment of the circuit is necessary; the gain
can be adjusted to unity by simply adjusting the 50 kohm
potentiometer that is part of R2. Any OP‑97 type of op‑
amp can be used in the circuit, such as the LT1097 from
Linear Technology or the AD705 from Analog Devices,
both of which offer pA bias currents, µV offset voltages
and are low cost. The input terminals of the op‑amps and
the photodiodes are connected in the circuit using Kelvin
connections to help ensure the accuracy of the circuit.
The next two circuits illustrate how the HCNR200/201 can
be used with bipolar input signals. The isolation amplifier
in Figure 18 is a practical implementation of the circuit
shown in Figure 14b. It uses two optocouplers, OC1 and
OC2; OC1 handles the positive portions of the input sig‑
nal and OC2 handles the negative portions.
Diodes D1 and D2 help reduce crossover distortion by
keeping both amplifiers active during both positive and
negative portions of the input signal. For example, when
the input signal positive, optocoupler OC1 is active while
OC2 is turned off. However, the amplifier controlling OC2
is kept active by D2, allowing it to turn on OC2 more rap‑
idly when the input signal goes negative, thereby reduc‑
ing crossover distortion.
Balance control R1 adjusts the relative gain for the posi‑
tive and negative portions of the input signal, gain con‑
trol R7 adjusts the overall gain of the isolation amplifier,
and capacitors C1‑C3 provide compensation to stabilize
the amplifiers.